Introduction to the James Webb Space Telescope
The James Webb Space Telescope (JWST), launched in December 2021, is a groundbreaking astronomical observatory that surpasses the Hubble Space Telescope. Built through collaboration between NASA, ESA, and CSA, it features a 6.5-meter primary mirror and advanced infrared technology. JWST’s enhanced capabilities allow it to study distant galaxies, star formation, and exoplanets in unprecedented detail. The telescope aims to explore the early universe and answer fundamental questions about cosmic origins, marking a new chapter in space exploration and scientific discovery.
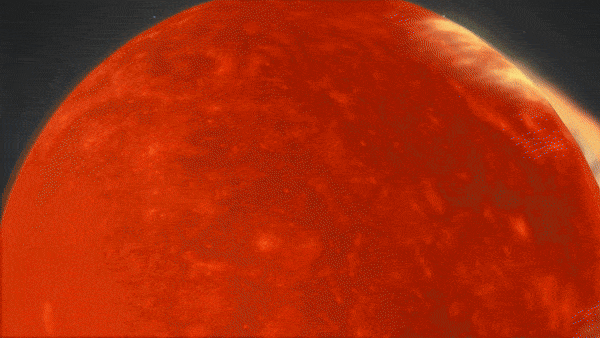
What is a Supernova?
A supernova is the explosive death of a star, releasing immense energy. There are two main types: Type I occurs in binary systems when a white dwarf accumulates material from a companion star, while Type II happens when massive stars collapse after depleting their nuclear fuel. Supernovae are crucial for spreading heavy elements throughout the universe, enriching galaxies with materials needed for new stars and planets. These explosions can also trigger the formation of new stars through shock waves, making them essential to cosmic evolution.
Introducing Supernova Hope
Supernova Hope emerged as a remarkable astronomical discovery, revealing significant insights into cosmic events that transpired approximately 3.5 billion years ago. This supernova is classified as a type II supernova, characterized by its explosion resulting from the gravitational collapse of a massive star. The remnants of this stellar explosion provide a unique window into the evolution of galaxies, offering valuable data regarding the lifecycle of stars and their contributions to the chemical enrichment of the universe.
One of the critical characteristics of Supernova Hope lies in its luminosity, which outshined entire galaxies at the height of its explosion. This brilliant light not only made it identifiable but also allowed astronomers to study its spectral properties in detail. The light emitted from Supernova Hope contains essential information about the elements forged during its explosion, contributing to the wider understanding of stellar nucleosynthesis. Much of the heavy elements observed in the universe today originated from supernovae like Hope, and studying its remnants enhances our comprehension of galactic environments where new stars form and evolve.
The discovery of Supernova Hope holds significant implications for astronomical studies focused on galaxy evolution. By analyzing the aftermath of supernova explosions, researchers can gain insights into the processes that influence star formation and the distribution of elements in various galactic structures. Furthermore, Supernova Hope exemplifies the role of such events in shaping the cosmos and influencing the gravitational interactions between galaxies. Thus, the classification and investigation of this supernova not only enrich the scientific community’s understanding of stellar life cycles but also illuminate the paths through which galaxies develop over billions of years.
The Role of Gravitational Lensing
Gravitational lensing is a fascinating astrophysical phenomenon that occurs when a massive object, such as a galaxy cluster, distorts the fabric of spacetime due to its gravitational influence. This warping effect can magnify and sometimes multiply the light from more distant cosmic objects, allowing astronomers to observe them in greater detail. The phenomenon is grounded in Albert Einstein’s General Theory of Relativity, which asserts that massive bodies can bend the path of light as it passes nearby. In essence, the more massive the object, the greater the curvature, resulting in a lensing effect that can reveal distant galaxies and other celestial phenomena otherwise too faint to detect.
The significance of gravitational lensing has become increasingly evident in modern astronomy, especially with the advent of advanced observational tools like the James Webb Space Telescope (JWST). The JWST capitalizes on this natural magnification, providing astronomers with new insights into the early universe and distant supernovae, such as Supernova Hope. By studying the light that has been bent and amplified by intervening mass, researchers can analyze the properties and behaviors of these far-off cosmic events, gaining critical information about their origins, chemical compositions, and their role in the evolution of the universe.
In relation to Supernova Hope, gravitational lensing has allowed astronomers to examine this explosive event with unprecedented clarity. The lensing effect not only enhances the brightness of the supernova but also reveals details regarding its environment and the surrounding celestial structures. Essentially, it acts like a cosmic magnifying glass, enabling scientists to speculate about the physical laws operating in the universe and enhancing our understanding of stellar explosions. As such, gravitational lensing plays a pivotal role in modern astrophysics, shining light on the enigmatic processes that govern the cosmos.
Hubble Tension: The Cosmological Dilemma
The Hubble tension represents a significant issue within modern cosmology, rooted in discrepancies between distinct methodologies for gauging the universe’s expansion rate, traditionally quantified by the Hubble constant. This constant serves as a critical index for understanding cosmic expansion, providing insight into the rate at which galaxies are receding from one another. Two primary approaches to measuring this constant yield contrasting results: one based on observations of nearby supernovae and Cepheid variable stars, and the other reliant on measurements of the cosmic microwave background (CMB) radiation from the early universe.
The tension arises primarily from the persistent gap in values produced by these methods. Direct measurements, which involve observing the cosmic expansion in the local universe, suggest a Hubble constant of approximately 73 kilometers per second per megaparsec. Conversely, data derived from the Planck satellite, which analyzes the CMB, indicates a lower value of about 67 kilometers per second per megaparsec. The implications of this discrepancy are profound, hinting at a more complex and nuanced understanding of the cosmos than previously anticipated.
To address this cosmological dilemma, scientists are undertaking comprehensive investigations to ascertain the roots of the tension. The conflict raises pivotal questions regarding our comprehension of dark energy, the enigmatic force propelling the acceleration of cosmic expansion. Furthermore, the Hubble tension suggests that there may be gaps in our existing models of the universe’s evolution or that previously unidentified factors may influence our estimations of cosmic distances.
As researchers analyze data from advanced observatories like the James Webb Space Telescope, there is hope that new findings may illuminate this critical issue. In pursuing an understanding of the underlying causes of the Hubble tension, the field of cosmology may inch closer to resolving long-standing mysteries concerning the expansion of the universe and its underlying physics.
JWST’s Contribution to Resolving the Hubble Tension
The measurement of the Hubble constant, which describes the rate of expansion of the universe, has been a subject of significant contention within the astrophysical community. The discrepancy between various methods of measurement has led to what is famously referred to as the Hubble tension. The James Webb Space Telescope (JWST) has emerged as a crucial instrument in this debate, particularly through its observations of gravitationally lensed supernovae. By capturing these extraordinary cosmic events, JWST provides an innovative methodology for determining the Hubble constant with greater precision.
Gravitational lensing occurs when the gravitational field of a massive object, such as a galaxy, bends the light emitted by a more distant object. This effect can magnify and distort the appearance of galaxies and supernovae, making them more observable from Earth. JWST’s advanced infrared capabilities allow it to peer into regions of the universe that have previously been difficult to study, capturing the light from these lensed supernovae. This observational data can be used to derive distances more accurately, potentially leading to a more unified understanding of the Hubble constant.
The implications of JWST’s data are profound. As we analyze the light curves and spectra from these gravitationally lensed supernovae, researchers aim to provide better estimates of the Hubble constant that could reconcile the differing measurements obtained through various methods, such as Cepheid variable stars and cosmic microwave background observations. The potential for a resolution to the Hubble tension could be a pivotal milestone in cosmology, enhancing our understanding of the universe’s expansion rate and its fundamental structure.
Ultimately, the observations and data derived from JWST will not only contribute to addressing the Hubble tension but also propel future research in cosmology, ensuring that our comprehension of the vast cosmos continues to advance.
Brenda Frye and Her Team’s Discoveries
Brenda Frye, an accomplished astronomer from the University of Arizona, has played a pivotal role in the groundbreaking discoveries made by the James Webb Space Telescope (JWST). Her research team’s identification of three distinct points of light in a critical JWST image has led to the momentous discovery of Supernova Hope. This finding is not merely a testament to technological advancement but also illustrates the collaborative nature that is increasingly characteristic of modern astronomical research.
Upon examining images captured by the JWST, Frye’s team utilized advanced data analysis techniques to differentiate between various celestial objects. They were particularly focused on what had been observed as faint glimmers in the depths of space. By employing sophisticated algorithms and cross-referencing other astronomical data, the researchers were able to confirm that these three points of light were not mere artifacts of the imaging process but genuine astronomical phenomena. This marked a significant breakthrough in the identification of the remnants of an exploded star, providing valuable insights into its composition and energy dynamics.
Collaboration was key in this remarkable achievement. Frye’s team worked in conjunction with multiple research groups, pooling expertise from a diverse range of scientific disciplines. The pooling of knowledge and skills not only expedited the analytical process but enriched the interpretation of the JWST data. Such cooperation among institutions is integral to overcoming the challenges present in modern astrophysical research, allowing teams to tackle complex questions about the universe collectively.
The discovery of Supernova Hope stands as a significant milestone in our understanding of stellar evolution and the lifecycle of stars. As Frye and her team’s work continues to unfold, it underscores the importance of teamwork, shared goals, and interdisciplinary collaboration in advancing astronomical inquiry and expanding our knowledge of the cosmos.
Follow-up Observations and Future Research
Follow-up observations are critical in the study of astronomical events, particularly for phenomena such as Supernova Hope, which was recently observed by the James Webb Space Telescope (JWST). These additional observations, often conducted using Earth-based telescopes, serve to validate the initial findings made by JWST and provide deeper insights into the implications of those findings. Advanced ground-based instruments, such as the Atacama Large Millimeter/submillimeter Array (ALMA) and the Gran Telescopio Canarias (GTC), offer complementary data that enhances researchers’ understanding of the supernova’s evolution and its impact on surrounding cosmic structures.
Through these follow-up observations, astronomers can monitor the temporal evolution of Supernova Hope, allowing for a thorough examination of its brightness, chemical composition, and the dynamics of its shockwave. Such details are essential for corroborating data acquired from the JWST and for addressing outstanding questions regarding the supernova mechanism itself. Furthermore, by observing how Supernova Hope interacts with its surrounding environment over time, astronomers can glean insights into the lifecycle of massive stars and the role they play in cosmic nucleosynthesis.
Looking ahead, future research endeavors will likely focus on leveraging both JWST’s capabilities and ground-based telescopes to explore a wider range of supernova occurrences across different cosmic eras. The integration of findings from the JWST, with its infrared sensitivity and resolution, alongside advanced spectroscopic observations on Earth, holds the potential to unlock new understandings of the universe’s expansion and elemental formation processes. Ongoing collaborations between researchers utilizing these diverse observatories promise a continued progression in unraveling the complexities of supernovae like Supernova Hope and their contribution to galactic evolution.
Conclusion: The Promise of JWST
In recent years, the James Webb Space Telescope (JWST) has emerged as a groundbreaking tool in the field of astronomy, providing insights that redefine our understanding of the universe. The discovery of Supernova Hope exemplifies the monumental capabilities of this advanced telescope. Through high-resolution observations, JWST has captured details of supernova explosions that were previously elusive, offering a glimpse into the life cycle of stars and the processes leading to their spectacular demise. The findings from this celestial event illustrate the importance of studying cosmic phenomena at various wavelengths, showing how JWST’s infrared capabilities can unveil the hidden aspects of stellar evolution.
The implications of these discoveries extend far beyond individual supernovae. They enhance our comprehension of the chemical enrichment of galaxies and the role of supernovae in star formation. As these cosmic events distribute heavy elements into space, they contribute to the material that forms new stars and planets, forging a continuous cycle of creation and destruction that shapes the universe. The data collected on Supernova Hope not only broadens our knowledge of these magnificent explosions but also sets the stage for future inquiries into other astronomical objects and events.
As JWST continues its mission, it promises to deliver even more groundbreaking discoveries that will further illuminate the complexities of the cosmos. The advancements in technology that allow for unprecedented observation capabilities ensure that astronomers can explore nebulas, distant galaxies, and exoplanets with incredible detail, fostering a deeper understanding of our place in this vast universe. The significance of JWST’s contributions cannot be understated; it is poised to transform our knowledge of space, offering new avenues for exploration and discovery that will keep the scientific community engaged for years to come.